
The Robots Within
The Koç University Rahmi M. Koç Medal of Science was created to inspire and encourage scientific progress. The award recognizes successful, pioneering scientists educated in Turkey who have contributed to universal knowledge either domestically or abroad. Now in its third year, in 2018 the medal was awarded to Metin Sitti for his globally influential work and contributions to science, engineering and medicine. We met with Metin Sitti to talk about the groundbreaking research for which he received the award, as well as his ongoing work and future research plans.
KURIOUS – As a researcher, you have worked on many different projects and made important findings. The medical robots at the center of your latest research are currently very popular. We assume you are not the first to work in this area – we’ve certainly found many examples in science fiction literature and cinema. What makes your work so unique?
Metin Sitti – Medical robots are not a new thing. There are very advanced commercial medical robotics systems, especially in laparoscopic surgery, or prostate, orthopaedic, brain and heart surgery. These are large, wired robots that work outside the human body and are the product of research conducted since the 1980s. The particular area I work on includes wireless micro- or nanorobots which can enter the human body and do surgery or deliver medicine, just like the ones in Fantastic Voyage. They can be easily and painlessly placed into the body either by being swallowed or injected, they can access very small and narrow regions because they are wireless, and they are pain-free and do not require the patient to be sedated. They are the future of medical technology. But of course, wireless miniature medical robots are still very new: they’ve only been around for about ten to fifteen years and there are other groups working on this area in Japan, Europe and the US. As for why our work is unique, we recently completed an important progressive step for these small devices. As well as being wireless, we’ve now designed and manufactured them using soft materials – something like a soft and flexible rubber band. We place magnetic particles in an elastic polymer and control them remotely by changing their shape from outside the body. The robots can change shape, navigate their way around the body and release medicine. We call these, externally controlled soft robots. They are not at all invasive and do not harm the body. Those large robots we mentioned earlier are quite risky because the slightest mistake can severely harm a patient. These, on the other hand, are harmless and can reach very different areas because they are soft and tiny.
How small exactly are these robots?
Currently, the first prototypes are just a few millimeters across, about four millimeters. We are trying to make them even smaller and scale them down to only tens of micrometers. Today’s catheters are also miniaturized to a scale of millimeters, but they have the problem of being wired – this means they are restricted in terms of where they can be used and can also pose a risk to the body. Even at the size of millimeters our work is unique because our devices are wireless and can be inserted into the body with the same medical functions of a wired device. They can navigate around inside the body and conduct medical procedures – that’s why these small, soft robots are our most influential work.
How do the robots move around when inside the body? Are they autonomous? How do they know where to go and what to do?
Generally, the most significant issue with medical devices is safe. We are very good at safety because our robots are soft-bodied, but as you mention, there is the question of how do we know where to go, who decides and who is in control? First of all, we have to know where we are inside the body, so the patient must be inside a machine which can show a physical image of the body, such as an ultrasound, X-ray or MRI. Because the robot is so small, we use an imaging device like this to let us know what’s going on inside the body and monitor the robot’s location. The doctor uses a monitor to follow where the robot goes and give it instructions. And although there is some risk while the robot makes its way around the body, the doctor is in control of the robot the entire time. Once the doctor plots a route, the robot navigates autonomously but must be followed visually at all times. The human body is extremely complicated so it is important to visually track it or it might end up in the wrong place or you might lose it. For the sake of both the process and the patient’s safety, it is always better to give control to someone who has extensive knowledge of the human body and an excellent grasp of the entire procedure.
Is the primary function drug delivery?
To a point, yes. The soft robot’s main feature is that we can change its shape remotely using a magnetic field. A sack on the robot opens when its shape changes which releases the drugs. The first application of this technique might be with cancer therapy, specifically chemotherapy. Currently, chemotherapy involves swallowing or injecting drugs to distribute them throughout the body, but in fact, 95% of cancer medicine fails to reach the correct destination when it is administered, causing side effects. This is why targeted cancer drug delivery is so highly sought after and why drug companies are working very hard to achieve this. In our study, we are trying to deliver a large dose to the correct location and release it locally to try and minimize side effects – this would offer a very important advantage. Doing this also increases the actual effect of the drug because it is released in the correct tissue at the highest dosage. This is the robot’s main function. Its secondary function is as follows. Let’s say you have abnormal bleeding in a blood vein or the brain or the cardiovascular system. We can send the robot to the site of the bleeding and have it change shape into a plug to patch the vein. The bleeding stops and if the conditions return to normal the robot can transform back to its original shape and relocate itself. In this way we can open and close different channels in the body according to a site-directed fashion. We can also heat the robot externally to give it a third function. The robot contains magnetic particles which heat up when you hit them with rapidly alternating magnetic fields. Cells begin to die at around 45C, so you can attach robots to cancerous tissue and treat them by heating the robots. This kind of treatment is called hyperthermia and is a form of chemotherapy.
How does the body discard the robots once they finish their jobs? Are there no side effects?
We should first answer the question how to get the robots into the body? We can do this in several ways according to where they need to be. For example, if our target is the digestive system they are swallowed. But they need to be injected if our aim is the cardiovascular system or the brain. Therefore, the insertion system changes according to the disease or the targeted location. But there are many problems to consider when putting robots inside a body. 1) the material used in manufacturing the robot must be compatible with the body’s cells. If the body doesn’t like the material it might react aggressively and create toxic side effects. This is a critical problem, so we manufacture our soft robots using materials the body will like. 2) The body’s immune system will attack the robots just like any biological or synthetic intruder. Therefore, the materials we use must also be friendly, or compatible with the immune system. If this is done correctly the robot will not provoke negative side effects. Finally, problems may arise if there is something wrong with the instructions given to the robot or if something goes wrong with its autonomous guidance. By performing procedures under supervision of a doctor, we can reduce such problems down to very small rates, close to 0%. So, once the robot or robots (because sometimes we will use more than one) is finished, what next? How do we get them out and what happens if we leave them inside? At this point, we have two solutions. Millimeter-size robots are magnetic so can be collected and removed using a magnet and catheter. What we’d rather do, and the method which yielded positive results in initial studies, is to have the robot dissolve completely inside the body. The critical thing is to ensure they dissolve over time because if they dissolve immediately you will lose the robot before it can finish its job.
How do you think this discovery will contribute to science? Which science fields will it affect later? What kind of projects can it lead to?
This technology is very interdisciplinary. Designing, manufacturing, controlling and operating such a robot requires different practices and expertise. To begin with, you need an understanding and knowledge of materials science because all the materials must be selected and produced correctly. It is essential your team includes someone who knows about materials science, chemistry and polymer chemistry. A good knowledge of robotics is also necessary. Robotics means you need an extensive knowledge of electrical and electronics engineering, mechanical engineering and programming. Then, besides being able to inspect and control the robot’s navigation you need to know physics and fluid dynamics. And of course, you can’t use these robots to conduct medical procedures without knowledge of physiology and medicine. What are the actions of that physiological system, what type of cells are there, what kind of reactions go on inside those cells, is there fluid flow or movement, and are the organs active? For that reason, you need a first-class knowledge of anatomy. So our team includes researchers from virtually every field gathered into one group. With any new scientific discovery you are making a major contribution to almost all fields of science. We are discovering new and novel materials. For example, the shape-changing materials of these soft robots are fairly new to science. The way we track them medically, how we insert and remove them: all this requires new scientific methods. These robots are so small (almost nano size) that we can manufacture and release them into the body in hordes of hundreds or even thousands. Of course, they need to be collectively controlled from outside, so we need to understand collective systems. We look at animals, from as small as tiny bacteria, to see how other small animals operate both independently and collectively: we learn so much from nature. Scientifically speaking, we build robots which learn from nature that are inspired by nature. For instance, this soft-bodied robot is a worm, but at the same time it is another type of soft-bodied jumping animal, or a drifting jellyfish or a swimming sperm. Nature has inspired many of our ideas. What is even more interesting is that while being inspired by nature helps you build new robots, actually building the robots also helps you better understand nature. Pursuing new medical applications, better understanding nature and designing new materials and methods inspires, and will continue to inspire, major inventions.
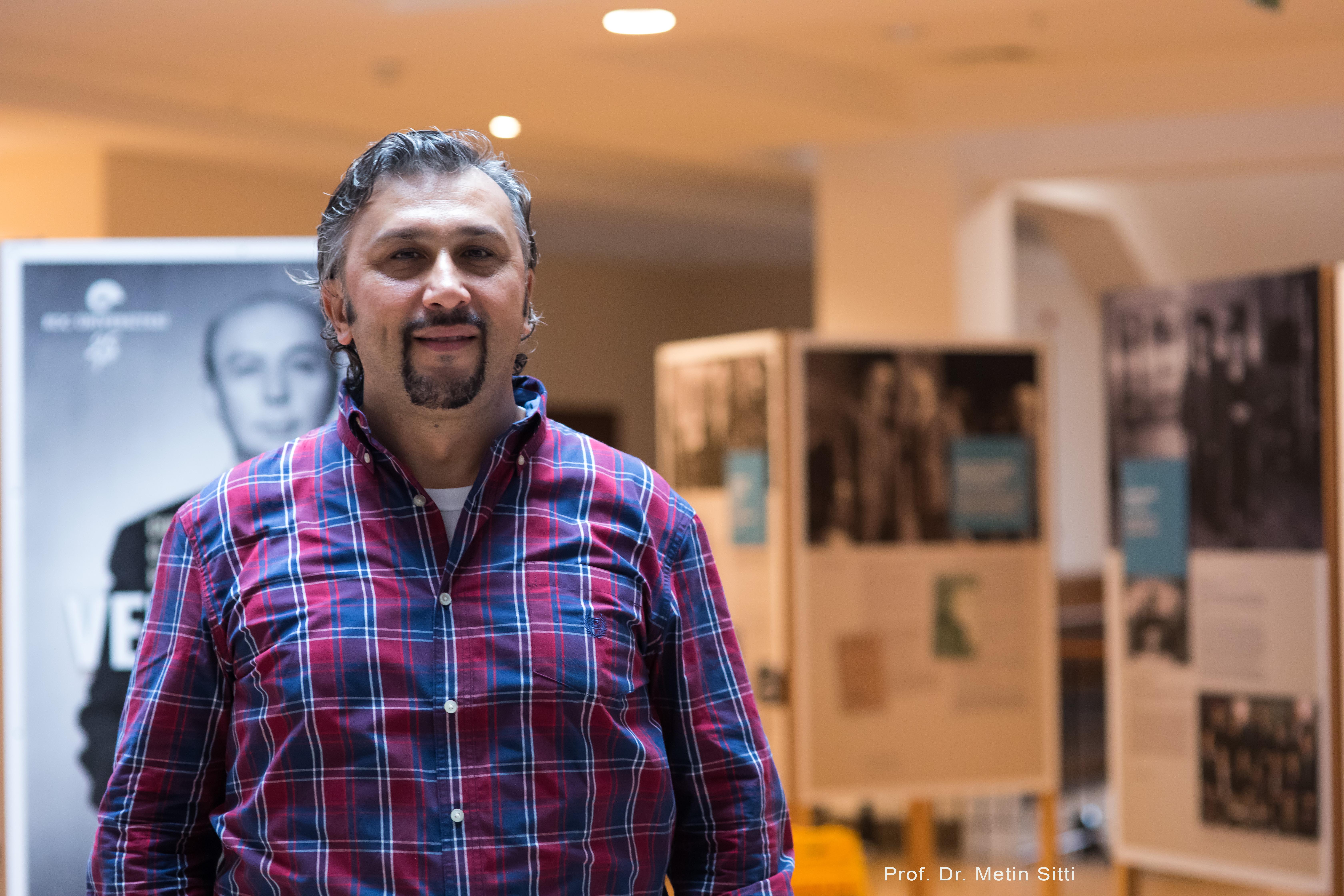
In a way this is a kind of biomimicry, in which you mimic the behavior of animals. I understand you also have another very different project: to develop robots by harvesting human muscle cells and adhering them to robot limbs. How do you picture the future? What awaits us twenty to thirty years from now?
There are two or three choices in terms of materials. The first is to manufacture everything synthetically, such as elastic or magnetic materials. Additionally, when we’re down to very small sizes like blood veins with diameters of 6-8 microns, a robot has to shrink to the size of a red blood cell in order to penetrate the veins. It is currently very difficult to synthetically produce robots on a cellular scale: some methods exist but they are not as efficient as biological systems. Therefore, instead of a fully synthetic production, we thought ‘why don’t we use real cells in the robots?’ Mobile living cells such as drifting bacteria, microorganism called algae or even our muscle cells are already mobile within the body. We also host about 1.5 kilograms of bacteria in our gut, which are mobile and live symbiotically with us. We combine these micron-sized organisms with synthetic drugs and synthetic materials – this is why our project includes the word ‘cyborg’. Biological systems are self-motile and driven by chemical energy, so there is no need to feed them with external energy. But when you build synthetic robots you need to provide energy for everything because there is no internal energy source. When you use a cell, on the other hand, it can be mobile for hours or days with the help of the cell’s own chemical energy. The beautiful part is, cells are aware of the pH, temperature, chemicals and oxygen found in their surroundings. This is why we also use cells as sensors. In the past, people have built carriages and used horses for transportation, or used the olfactory senses of dogs and pigs to find certain materials and mushrooms. Throughout history we’ve used animals for various purposes, and in the same way we are now using their micrometer-size versions. We produce small robots using the sensory, motor and chemical properties of micron-sized biological cells. And so the robots of the future will not be entirely synthetic, but will bring together advantages of both the synthetic and the natural: in other words, cyborg.
This reminds us of Ray Kurzweil’s book, Singularity is Near, where he says there will be no war between humans and machines but instead there will be a singularity in which they merge.
When I present these subjects at medical conferences, doctors ask ‘will robots replace doctors?’. In the future a robot could technically replace a surgeon, but this is not our objective. In fact it isn’t the robot’s objective either. The goal is always to create machines to help humans. Robots have started to replace humans in certain factory production lines, is that good or bad? It is good if you use it correctly and wisely, but as I said, in general it is better to have robots work with people rather than replace them. This is also our objective: we aim to produce medical robots that work with the doctor, help the doctor and make the doctor’s job easier. These robots can help in drug delivery, surgical operations and diagnosis. Of course, early diagnosis is very important. And so, our dream is to develop systems in which robots can be used to constantly roam around and monitor our bodies, notifying the doctor when they sense any abnormal changes. Maybe in the future, as you mention, robots will become an internal part of us.
Patients will always prefer to have a real doctor. Even though we may have successful or talented robots, people still require compassion.
Of course, compassion and trust are important but besides this there is another ethical debate. If an autonomous car causes an accident who is responsible? The car’s manufacturer? The person who wrote the algorithms? Or the driver? The same problems apply here. Who is responsible when something goes wrong? If the robot causes an accident while it is not under the guidance of the doctor, then you will need to blame the robot. After all, the point of putting a doctor in control of everything is so that the doctor has legal, psychological and technical responsibility, while also fulfilling certain human needs for the patient. Maybe future robots will be capable engaging emotionally with patients but ultimately, it is better not to lose the human dimension.
You are in charge of a large department at the Max Planck Institute, a very important place. Do you recommend young scientists to broaden their interests and study interdisciplinary subjects?
Almost all studies have become interdisciplinary. Interdisciplinary teams advance quicker in terms of making new inventions. But this does not mean you need to simultaneously learn biology, materials, chemistry, medicine, etc. In fact, it is essential to complete a focused undergraduate education in a basic field. Interdisciplinary studies become especially important in graduate, postgraduate and doctoral programs, as well as in research. Such students who are open to innovations, new ideas and other disciplines become more successful. Of course you need to study hard, too. I received my robotics education by completing a double-major in physics and electrical and electronics engineering, topped off with a postgraduate in electrical engineering. But I didn’t stop there. I continue to learn all the time, including biology, medicine, materials science and many other disciplines. My education taught me a very important ability during my education: learning how to learn. My advice to students is to get a very solid, extensive knowledge of a core field, move on to newer fields in addition to that education, and learn how to learn. As you place new knowledge on your basic knowledge, you will become much more successful. Of course, you also need to learn teamwork. In the end, you may know everything up to a certain depth but you will need experts in biology, materials science and robotics to come together in multidisciplinary teams. Students who have managed to build a certain repertoire but who have also adopted and understood teamwork personally and culturally can become flexible students and are more likely to complete very successful studies.
You were awarded the Rahmi M. Koç Medal of Science. Receiving a reward is certainly a prestigious achievement, but does it offer any other benefits to researchers?
The most important advantage of medals or awards is that they increase the recipient’s visibility. These prizes are excellent tools for making a researcher better known in Turkey or abroad. People learn about you, students hear about you and researchers recognize you – this opens a lot of doors. Students come to study with you or researchers approach you for collaborative studies. In this way it increases the influence of your scientific efforts. Until now, I had received many awards in foreign countries but none in Turkey. The reason why the Rahmi M. Koç Medal of Science is so special for me is that it is the first time I have won an award in Turkey. This makes me very happy. I had never severed my ties with Turkey, but thanks to this medal I will be able to participate in more studies here, train students, and find opportunities for collaborations. By doing collaborative studies here, I hope to boost people’s contribution to science.
All in all, we can’t deny there are benefits to the people of our country when they have broader horizons and understand science better.
My personal philosophy has always been not to follow the same direction as everyone else. Currently, young people worry about getting a footing abroad as soon as possible. But if you look at successful countries, they are all trying to find ways to keep talented and qualified people at home. We need to be pursuing science and creating new technology here in Turkey. Letting it all go abroad is dangerous for the country’s future. Of course, this doesn’t mean that everyone should stay here and no one should go anywhere. We can do science here as much as is possible and students should stay as long as they can: and those who leave – including teachers – should try to return. Everyone asks me why I came to Europe from the US when most people are trying to get to the US. I wanted to be closer to Turkey – that was one of the most important reasons. And I did get closer. Collaborations with Koç University and this medal will of course contribute further to this. There are great benefits in supporting and creating collaborations here in Turkey. I also intend to bring contacts and opportunities from abroad. Acting as a bridge is an important role for all of us. I mean, beyond personal success, it is always important to facilitate science, support successful studies and enable students to do research in our country. Koç University does this very well, so I think it’s valuable to be supportive.
So, our last question… what important steps should be taken to fulfil young scientists’ most urgent needs, or what steps should be taken to support them?
I have seen many systems, from Japan to the US and Europe. The most important thing for the success of our young scientists is a good education and a free environment. That is exactly the time when a young person’s personality takes its final shape. It is also the period when their scientific curiosity, their future plans and their knowledge pool begins to take shape. Therefore, a free environment and a high-quality education are pivotal. It is important to create an environment that can maintain quality at a certain level while having both financial support and good educators. But besides this, opportunities created by universities are also important. For example, better research opportunities will increase the success of our young scientists in scientific research. Doctoral students are one of the most fundamental elements in research and so some of our good students should be persuaded to do their research in Turkey. To do this, it is imperative to have a research environment that will satisfy these students, as well as overseas opportunities and visits. We are considering joint doctorate programs to enable this. Students could do a doctorate here and at the same time visit the Max Planck Institute in Germany or an institution in the US, and participate in joint doctorate programs. To conclude, we have to create environments where these students can be happier and more successful.