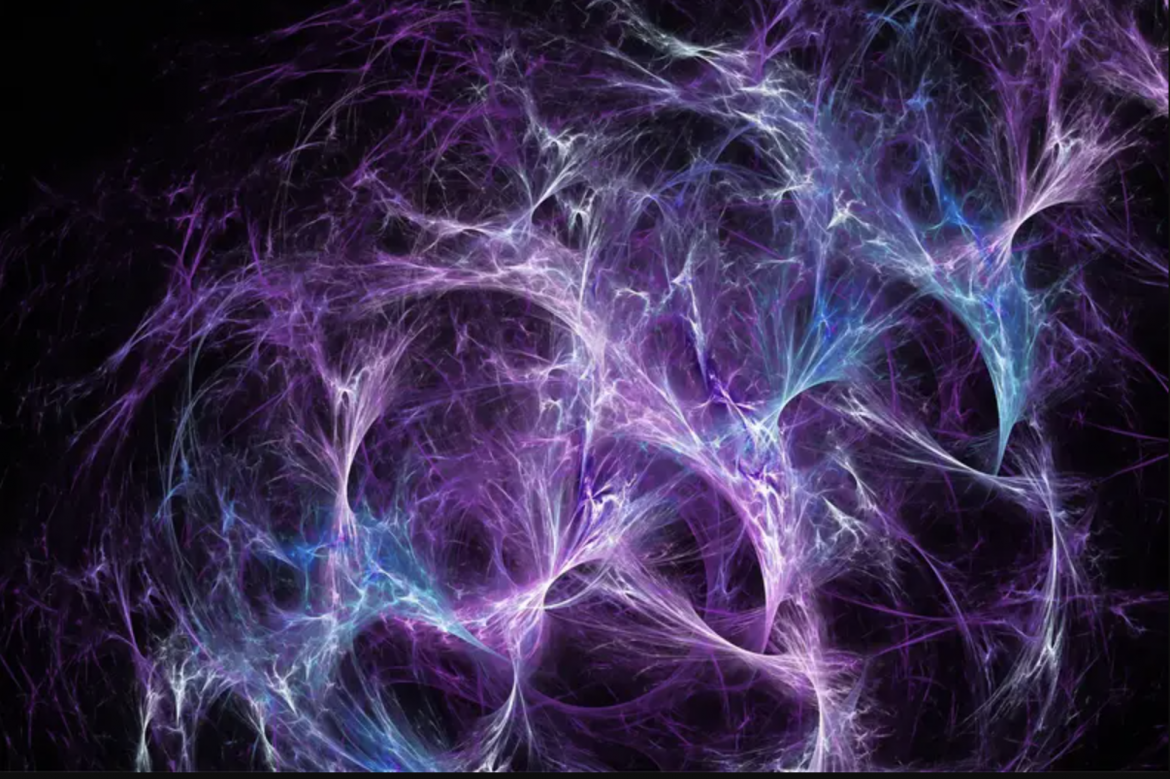
In Pursuit of Dark Matter
According to currently accepted cosmic theories, it is believed that the universe is composed of three components. “Ordinary matter” is the substance that makes up stars, planets, humans, and everything visible. However, this matter actually constitutes only about 5% of the total mass and energy of the universe. The remaining 95% is thought to be made up of two mysterious substances, both invisible and inherently unusual, but believed by scientists to play profound roles in the evolution and expansion of the Universe: “dark matter” and “dark energy.”
It is the matter that forms stars, planets, humans, and everything in the universe. It comprises protons, neutrons, and electrons, forming atoms and molecules. Although it is directly observable, ordinary matter accounts for only about 5% of the total mass and energy of the universe.
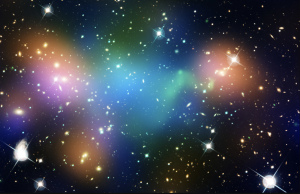
Dark Matter
In the mid-20th century, astronomers observed that the rotational velocities of galaxies were higher than what could be accounted for by visible matter alone. Calculations indicated that the stars, gas, and dust within galaxies were rotating at speeds that couldn’t be explained by the gravitational effects of observable matter. As a result, the existence of a form of matter that was invisible in the universe and different from normal matter began to be considered to explain the extra gravitational effects observed in galaxies. This form of matter was named “dark matter.”
Dark matter is a mysterious and invisible substance. It does not emit, absorb, or reflect light, making it undetectable by traditional electromagnetic radiation-based telescopes. However, its presence can be inferred from its gravitational effects on visible matter and galaxies. Dark matter constitutes approximately 27% of the total mass energy of the universe and plays a significant role in the formation and structure of galaxies and galaxy clusters.
Dark Energy
The discovery of dark energy occurred through astronomical observations made in 1998. Observers looking at distant galaxies and supernova explosions found that the expansion rate of the universe was slower in the past and surprisingly accelerated as time progressed. This discovery showed that the gravitational pull of the universe had an accelerating effect rather than a slowing effect. This acceleration could not be explained by the gravitational effects expected from the known normal matter and dark matter in the universe.
Astronomers realized that they needed a new type of energy to understand the cause of this acceleration. This new form of energy was named “dark energy.” Dark energy appears to be uniformly distributed in the empty space of the universe and is considered a mysterious form of energy that accelerates the expansion of the universe.
Similar to dark matter, dark energy is not a type of matter or particle; instead, it is a property of space itself. It acts as a counterforce to gravity and causes the universe to expand at an ever-increasing rate. Dark energy constitutes approximately 68% of the total mass-energy content of the universe.
Ordinary matter, dark matter, and dark energy, these three components form the best understanding of the current composition of the universe. However, the nature of dark matter and dark energy remains one of the greatest unresolved mysteries of modern astrophysics and cosmology. Scientists continue to study and research these mysterious components to gain a deeper understanding of the fundamental workings of the universe.
In Pursuit of Dark Matter
Although its existence is inferred from gravitational effects on visible matter, the exact nature of dark matter is still unknown. Some of the main candidates for dark matter include:
Weakly Interacting Massive Particles (WIMPs)
WIMPs are hypothetical particles that interact very weakly with normal matter, making them extremely difficult to detect. It is predicted that WIMPs have a mass similar to the Higgs boson.
The significance of WIMPs, if they are real, is that they must possess the right properties to explain why there is so much dark matter in the universe. In the early universe, they were abundant, but as it cooled and expanded, they became very rare, leaving enough dark matter that we observe today.
Scientists use advanced experiments like DARWIN and LUX, which involve extremely cooled xenon, to capture these hard-to-detect particles. In these experiments, detectors are placed deep underground to reduce the noise from cosmic rays by approximately 10 million times. Then, they wait for a WIMP to interact with xenon atoms inside the detector.
Researchers believe that when a WIMP collides with a xenon atom, it creates a flash of light that releases electrons. Photomultiplier tubes (PMTs) detect this and register the electrons through an electric field. If this happens at the center of the detector, it could be the signal scientists hope to see.
The challenge here is designing detectors with enough sensitivity to detect the tiny amount of energy that WIMPs would leave when interacting with normal matter. Additionally, they are investigating theories like Supersymmetry, which suggests the existence of WIMP-like particles.
If scientists can detect WIMPs, it would be a significant discovery that will help us understand the enigmatic dark matter that shapes our universe.
Axions: Axions are hypothetical particles that have no electric charge and behave like waves due to their very small mass. They are important in solving problems in nuclear physics and explaining the abundance of matter compared to antimatter in the universe. Axions were likely produced in large quantities during the Big Bang.
Scientists are conducting experiments using sensitive equipment like sensors and antennas to detect axions. They are also searching for them in the halo of our Galaxy and in the Sun. Some experiments like CERN’s Axion Solar Telescope and IAXO focus on detecting axions produced inside the Sun. Others like ALPS and OSQAR use powerful magnets to find axions produced through photon conversion. Another experiment called ADMX aims to convert undetectable axions into microwave photons using strong magnetic fields.
While axions have not been directly detected yet, they hold great potential in helping us understand the mysteries of our universe.
Sterile Neutrinos: Neutrinos are incredibly lightweight, almost massless particles. However, recent discoveries have shown that they actually have a small mass. Sterile neutrinos are a special type of neutrino proposed to explain some interesting effects. Unlike normal neutrinos, they interact only through gravity and not through electromagnetic forces. Therefore, scientists consider them as potential candidates for dark matter, the mysterious substance that makes up a large portion of the universe.
Detecting sterile neutrinos is challenging because they have very small masses and interact weakly with other particles.
Sterile neutrinos are necessary to understand neutrino masses and can help us understand why there is more matter than antimatter in the universe. More research is needed to confirm their existence and unravel their role in the cosmos.
New and Significant Developments
While much of the research on dark matter remains purely theoretical, physicists are actively trying to search for signals or signatures of dark matter particles in various mass ranges using the characteristics predicted by proposed models. In this intriguing quest, future expectations can analyze how different dark matter candidates behave under the conditions of a specific dark matter model/theory. Creating more powerful and sensitive particle colliders than the LHC can also assist in the search for dark matter. A model that includes more types of dark matter with complex dynamics could also be proposed. Additionally, we need to collect and analyze more cosmological data. Further research on dark matter has the potential to lead us into a whole new era of physics, where we can successfully go beyond the standard model and revolutionize this field of study.
Euclid: In early July of this year, the European Space Agency (ESA) successfully launched the Euclid space telescope, initiating a new scientific mission toward the unknown with the aim of “exploring the composition and evolution of the dark Universe.”
After completing the initial tests and calibrations of Euclid’s instruments and systems, the telescope will spend six years observing about one-third of the sky to create a three-dimensional map of the Universe in space and time.
Euclid will attempt to reveal how dark matter behaves through its observations, explain how it is distributed in the universe, and measure gravitational lensing.
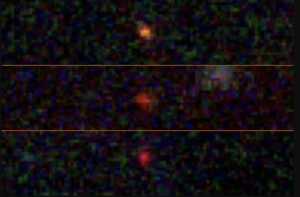
JWST: The James Webb Space Telescope (JWST) may have sighted a rare type of star, the existence of which is not even certain to astronomers. These star objects, known as “dark stars,” could have been fueled by the self-destruction of dark matter – the invisible stuff thought to make up about 85% of the matter in the universe – rather than by nuclear fusion. More evidence will be needed to confirm the candidate stars seen by JWST, but if they are real, the finding could change our story of how the first stars formed.
Researchers have not yet been able to prove that these objects are dark stars – only that their characteristics are consistent with them being dark stars or galaxies that host regular fusion-operating stars. Cosmin Ilie, a co-author of the study and an astrophysicist at Colgate University, says that JWST’s technology is adequate for this job. The only thing researchers need is more observation time. Ilie says, “We hope to find one of these dark stars within Webb’s lifetime.”
Hyper Suprime-Cam (HSC): Massive structures, like galaxies, bend the light passing through their regions through gravitational lensing, changing the appearance of background objects. The extra gravity created by dark matter, which cannot be directly observed, can be observed through gravitational lensing.
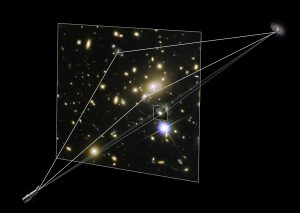
Devices like the Hyper Suprime-Cam (HSC) of the Subaru telescope in Hawaii allow measuring the discrepancies in the lensing of such large celestial bodies. With these results, indirect data about dark matter can be obtained.
Recently, researchers using HSC conducted measurements on 25 million galaxies, successfully measuring this very small effect with high precision. While the papers related to these measurements have not been published yet, the possibility of a finding that may require modifications to the current cosmological model is even more exciting.
The future of dark matter research is exciting, and as technology advances and new discoveries are made, we can expect a deeper understanding of this mysterious component of the universe. It is likely that dark matter plays a crucial role in unraveling the mysteries related to the formation of structures, the evolution of the cosmos, and ultimately the fundamental nature of the universe itself.
REFERENCES
- 1. https://www.esa.int/Science_Exploration/Space_Science/Euclid/ESA_s_Euclid_lifts_off_on_quest_to_unravel_the_cosmic_mystery_of_dark_matter_and_dark_energy
- 2. https://science.nasa.gov/astrophysics/focus-areas/what-is-dark-energy
- 3. https://scitechdaily.com/how-to-see-the-invisible-measuring-dark-matter-with-hyper-suprime-cam-reveals-discrepancy/
- 4. https://www.scientificamerican.com/article/jwst-might-have-spotted-the-first-dark-matter-stars/
- 5. https://bigthink.com/starts-with-a-bang/xenon-experiment-wimp-dark-matter/
- 6. https://www.space.com/gravitational-lensing-explained